Space Weather Projects at ISR
Researchers at BC's ISR participate in a variety of domestic and international projects involving solar-terrestrial physics, including studies of the solar wind, coronal mass ejections, and interplanetary propagation of geo-effective storms. These storms and similar disturbances can affect communication and navigation systems, particularly for active spacecraft, and the most powerful can affect ground-based systems including power grids.
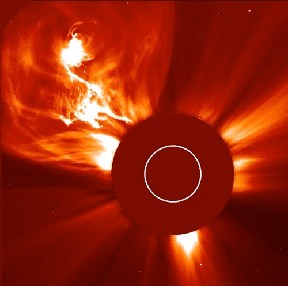
Space Weather
ISR personnel have worked for many years in collaboration with several government agencies, including the Air Force Research Laboratory (AFRL), NASA and the Navy, on programs involving solar-terrestrial research and heliospheric imager instrument development, data processing and data analysis. Our goals are to understand the origins and interplanetary (IP) propagation of disturbances that affect the geosphere, to support data processing and analyses from AFRL spacecraft missions such as the Solar Mass Ejection Imager (SMEI) and the Communication / Navigation Outage Forecasting System (C/NOFS) mission, and to help develop next-generation heliospheric imagers. The research utilizes many spacecraft and ground-based observations of solar, IP and geomagnetic phenomena and observations from the SMEI, STEREO Heliospheric Imagers (HIs) and other current and future imaging experiments.
A major portion of this work involves research using these data sets to address three scientific topics of current importance:
- The solar eruptive phenomena which lead to sporadic geomagnetic storms;
- Shock waves and other IP signatures of solar ejecta;
- The characteristics of IP disturbances which produce geomagnetic storms.
The most important goal of the studies is to utilize data sets related to solar ejecta and their IP manifestations to define the origins, geometry and propagation characteristics of geoeffective disturbances. Boston College ISR personnel have been at the forefront of such studies and, therefore, have been instrumental in supporting our government’s space weather programs.
Coronal mass ejections (CMEs – see Webb and Howard, 2012 for a review) and their associated phenomena are the key drivers of major space weather at Earth and throughout the heliosphere. Figure 1 shows a SOHO LASCO C2 image from 4 January 2002 of a CME showing detail in the ejected material. The solar limb Sun is represented by the white circle. Major CMEs inject large amounts of mass and magnetic fields into the heliosphere and, when aimed Earthward, can cause major geomagnetic storms and drive IP shocks, a key source of solar energetic particles. CMEs exhibit variable speeds, intensities and trajectories.
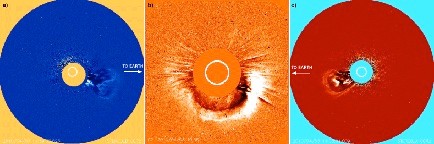
Figure 2 shows images of the same event (an Earth-directed CME in early April 2010) observed from three different viewpoints. The center (b) shows the perspective from SOHO LASCO along the Sun-Earth line where the CME appears as a halo. (A) and (c) show the same CME as observed by each STEREO spacecraft, separated in longitude by ~70° from LASCO at this time. The event appears in each COR-2 image as a limb CME directed towards the left (right) relative to STEREO-A (-B). The dramatic change in the appearance of the CME, with the only physical change being the viewing location, demonstrates the importance of perspective with respect to measuring CME properties and tracking its direction.
Currently, predicting when a CME will be launched from the Sun is very uncertain, and to better forecast and mitigate their effects it is necessary to detect and track CMEs from near the Sun to Earth with a heliospheric imager/detector. Without such monitoring, forecasters are blind to CMEs for 85% of the Sun-Earth distance and, thus, unable to accurately predict the time of arrival and trajectory of a CME, or the intensity and duration of the consequent geomagnetic storm.
Studies with SMEI and STEREO have proven that a heliospheric imager in Earth orbit and/or at suitable locations in space can provide early warning of ~1-3 days of Earth arrival of CMEs, the driver of major storms. AFRL, NASA and the Navy are involved in efforts to develop next-generation heliospheric imagers with the goal of providing an operational forecast system. ISR personnel support and analyze the data returned from the SMEI and STEREO HI experiments, and are supporting the development of next-generation imager experiments.
Select Publications:
Webb, D. and Howard, T. 2012, "Coronal Mass Ejections: Observations", Living Rev. Solar Phys. 9 (2012), 3. Link.
Point of Contact for this project is David F. Webb.
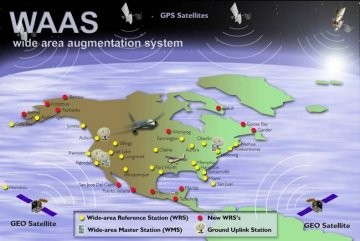
Space Weather Effects on GPS and WAAS
Space Weather describes the conditions in space that affect Earth and its technological systems. It is a consequence of the behavior of the Sun, the nature of the Earth’s magnetic field and our location in the solar system. The Sun is the major source of space weather on Earth. The features on the Sun reveal the active and turbulent nature of this highly magnetic and hot gaseous star.
Space Weather events such as solar flares, coronal mass ejections and solar radio bursts can have major effects on space-based technological systems including GPS and WAAS. Solar flares and CMEs induce geomagnetic storms that make the ionosphere unstable, resulting in rapid changes and strong spatial gradients. Solar flares travel at the speed of light; however, their effects on GPS receivers have been noted to begin approximately one hour later. CMEs travel a lot slower, typically reaching Earth in one to five days after the eruption from the Sun. CMEs are the most threatening of solar events.
Space Weather Effects on GPS
Space weather events, such as those described above, can affect the performance of groundand space based technological systems, with effects ranging from minor digital upsets, to severe power grid disruptions that can cause loss of service to millions.
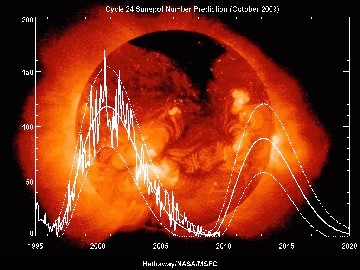
For GPS users, the impact of space weather can usually be characterized by disturbances in the ionosphere. The ionosphere is that part of the atmosphere that is ionized by solar radiation. It has practical importance because it influences radio wave propagation between space and earth. The ionosphere over the CONtiguous United States (CONUS) is normally well ordered with slow and predictable changes in the ionosphere. During a geomagnetic storm, however, rapid changes and strong gradients over distance can ultimately degrade GPS ranging measurements.
Very severe magnetic storms can also introduce ionospheric scintillation activity in the mid-latitude CONUS region. Scintillation is a rapid variation in the intensity of the satellite signal that can cause a GPS receiver to lose lock on the signal. If many satellite signals are affected by scintillation, it can cause GPS to lose the capability to provide positioning information.
Scintillation and the presence of large gradients, as illustrated in Figure 2, are a major concern for wide area ionospheric modeling systems such as the FAA’s Wide Area Augmentation System (WAAS).
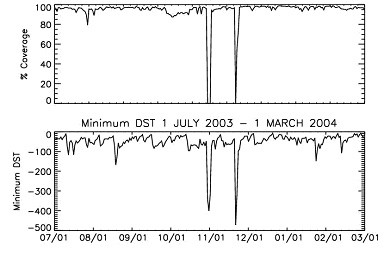
Space Weather Effects on WAAS
WAAS is a satellite based navigation system. It was designed by the FAA to become the future primary means of civil aircraft navigation. It was commissioned for approaches with vertical guidance (APV) in July 2003. What that means is that aircraft can be guided to 200ft above a runway without the help of an ILS (Instrument Landing System) – even in poor visibility conditions.
In the WAAS system, the standard GPS service is augmented with corrections for time, the GPS satellite orbits and the ionosphere. These augmentations enable the WAAS system to meet the very stringent aviation requirements for accuracy, availability and integrity. Quarterly performance reports have shown that the WAAS system generally meets or exceeds these requirements [http://www.nstb.tc.faa.gov]. The performance reports also reveal that one of the greatest challenges for WAAS is maintaining continuous APV availability during extreme geomagnetic storms.
Since WAAS was deemed operational, ISR has assisted the FAA in monitoring space weather effects on the WAAS system. The result of that analysis is primarily captured by Figure 3 where availability of APV service is limited under severe magnetic storm activity. The effects of less intense magnetic storms vary, with some inducing shorter regional losses of availability and others having little effect on availability.
Point of Contact for this project is Keith Groves.
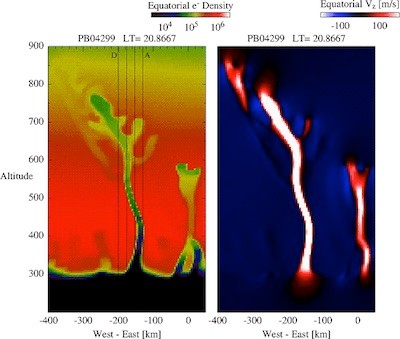
Space Weather Forecasting
Space weather is the consequence of the ever-changing output of the sun in energetic particles and electromagnetic radiation which impacts the near-Earth space environment and can affect human activities. Because of these effects we would like to be able to make predictions of when strong impacts might happen, so that system operators can make preparations to minimize damage.
The process of making these space-weather forecasts is much like the process of forecasting terrestrial weather: We first attempt to determine the current state of the space environment as accurately as possible using observations and measurements. Then the equations that describe how the plasma environment of the near-Earth space evolves and how it responds to stimuli are used to advance this state to make a prognosis of the state in the future.
One example of this kind of forecast deals with the occurrence of plasma irregularities in the ionosphere that can interfere with radio propagation and affect communication and navigation (GPS) systems. This interference occurs when naturally occurring waves in the ionosphere become large in amplitude and the ionosphere becomes turbulent, generally as the result of the nonlinear evolution of plasma instabilities.
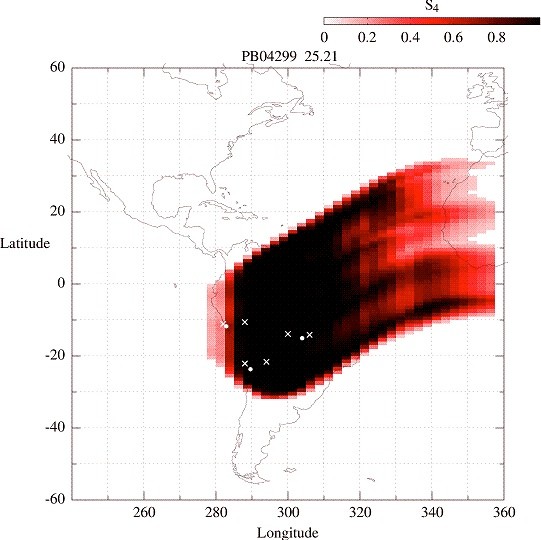
To forecast the development of this turbulence, the conditions within the Earth’s magnetosphere/ionosphere/thermosphere system are examined for susceptibility to the occurrence of these instabilities, which are then tracked by predicting the development of structure in the plasma density when they occur. When low-density structures of fast-moving plasma like the plume in Figure 1 occur, they generally are full of the irregularities that lead to radio scintillation.
From this structure, predictions of the strength of radio scintillation can be made, as shown overlaid on the map of the Americas in Figure 2.
The final stage of the forecasting process is validating the predictions by comparing them with subsequent observations made in the forecast time interval (see Figure 3: the red curves are the observations of scintillation strength as a function of time, the blue curves are the model predictions; each panel represents a different station in South America):
Such a model has been developed by ISR personnel to be used to help understand the phenomena of ionospheric irregularities (to deal with some of the fundamental problems of space plasma physics) and improve our techniques for forecasting space weather (to provide a practical application). It is currently running and being used at sites making space-weather predictions for commercial aviation, for Air Force satellites, and can be publically viewed at the NASA webpage.
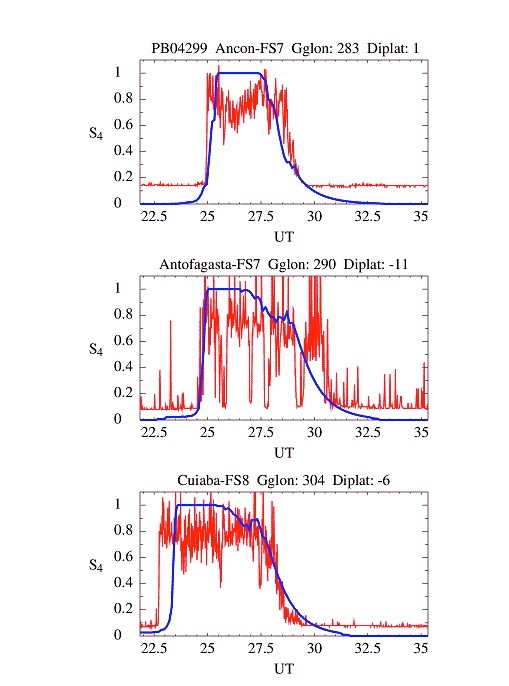
Publications:
Retterer, J. M. (2005), "Physics-based forecasts of equatorial radio scintillation for C/NOFS", Space Weather, 3, S12C03.
Retterer, J. M. et al. (2005), "Assimilative modeling of the equatorial ionosphere for scintillation forecasting: Modeling with vertical drifts", J. Geophys. Res., 110, A11307.
Kelley, M. C. and J. M. Retterer (2008) “First successful prediction of a convective equatorial ionospheric storm using solar wind parameters”, Space Weather, 6, S08003, DOI.
Retterer, J. M. and L. C. Gentile (2009) “Modeling the climatology of equatorial plasma bubbles observed by DMSP”, Radio Sci., 44, RS0A31, DOI.
Retterer, J. M. (2010) “Forecasting Low-Latitude Radio Scintillation with 3-D Ionospheric Plume Models: I. Plume Model”, J. Geophys. Res., DOI.
Retterer, J. M.(2010) “Forecasting Low-Latitude Radio Scintillation with 3-D Ionospheric Plume Models: II. Scintillation Calculation”, J. Geophys. Res., DOI.
Retterer, J. M. et al. (2010) “Modeling the low-latitude ionospheric electron density and plasma turbulence in the November 2004 storm period”, J. Atmos. Solar-Terr. Phys., DOI.
Su, Y.-J., J. M. Retterer, et al. (2009) "Assimilative modeling of equatorial plasma depletions observed by C/NOFS", Geophys. Res. Lett., 36, L00C02, DOI.
Kelley M. C., J. J. Makela, O. de La Beaujardière, and J. Retterer (2011) "Convective Ionospheric Storms: a Review”, Rev. Geophys., DOI.
Point of Contact for this project is John Retterer.